Author: 不想实名好害羞
Link: 知乎 – Copper Substituted Lead Phosphate’s Peculiar Memory Effect in Low-Field Microwave Absorption Source: Zhihu Copyright belongs to the author. For commercial reproduction, please contact the author for authorization. For non-commercial reproduction, please cite the source.
This article provides a straightforward interpretation of the entire content, addresses some questions from the community, and introduces some key terms. The article is open for casual redistribution and rewriting.
Key Terms to Understand in Advance:
- Microwave Absorption: When microwaves pass through a material, a part of their energy is absorbed by the material, known as microwave absorption. This can reflect the microstructure of the material.
- Electron Paramagnetic Resonance (EPR): EPR is a technique for precisely measuring unpaired electrons in a sample. In a magnetic field, electrons perform regular precession movements. When their frequency matches the conditions for EPR, they absorb microwave energy, thus detecting electron signals.
- Shielding Current: Superconductors can completely repel external magnetic fields, known as the Meissner effect in superconductivity. The current that generates this effect is called the shielding current. It circulates on the surface of the superconductor, maintaining zero magnetic field inside.
- Vortex: When a strong magnetic field is applied, it can break the complete pairing of electrons in a superconductor, forming a vortex-like current pattern, known as vortices. The movement of vortices reflects the excited state information of a superconductor.
- Bistability: When a system switches between different stable states, and the state depends on its history, it is called bistability. Simply put, it’s a memory effect.
- Glassy State: Glass is neither a solid nor a liquid; it is in-between, having the rigidity of a solid and the disorder of a liquid. A system in a glassy state has slow dynamics and takes a long time to reach equilibrium, hence displaying memory characteristics.
Simplified Explanation for Beginners: A new material called Copper Substituted Hydroxyapatite has shown an astonishing “memory effect!” This material was synthesized by a research team using a special chemical method. Its composition includes lead, copper, phosphorus, and oxygen (the detailed composition is not a focus for the simplified version). Importantly, it exhibits unique magnetic properties.
Researchers used a technique called Electron Paramagnetic Resonance to test this new material. This sensitive measurement method can detect weak magnetic responses of electrons in the material. The researchers applied an external magnetic field to the sample and irradiated it with microwaves. By analyzing the absorption of the microwaves, information about the movement of electrons in the sample was obtained. The tests revealed that when the external magnetic field was changed slowly, this new material exhibited a “hysteresis” phenomenon in its microwave absorption strength, meaning the absorption strength changes lagged behind the magnetic field changes. This lag showed a distinct “memory effect.”
What’s more intriguing is that if the researchers rotated the direction of the sample, this memory effect gradually “forgot” itself. Even stronger magnetic fields couldn’t reactivate it. However, if the sample was left untouched for two days, the memory effect miraculously restored itself! The researchers believe this unique memory effect originates from the special electronic conditions in the material. They successfully simulated and explained this memory effect using a “quantum lattice model.” The model suggests that the electrons in this material form a particularly fragile ordered state, akin to a glassy state, which can maintain its magnetic memory but can also be disrupted by rotation. The formation temperature of this ordered state is around 250 Kelvin. The specific mechanism still requires further study. This unique “memory effect” provides hope for potential applications of the material, such as in new types of magnetic memory storage materials.
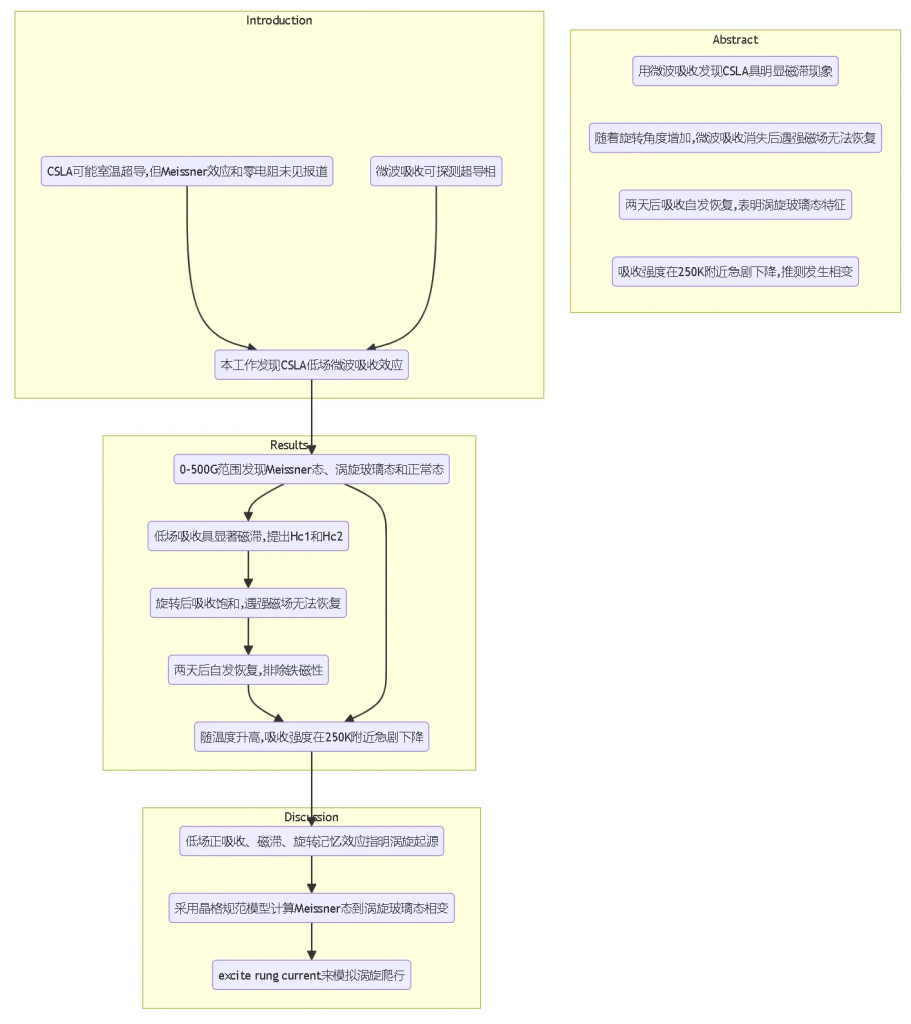
The paper discusses the superconducting properties of Copper Substituted Lead Phosphate (CSLA) at room temperature and atmospheric pressure, a claim made by Lee and others. The research pivots around using microwave absorption to detect possible superconducting phase transitions in CSLA, as the powder form of CSLA prevents conventional resistance and magnetization measurements.
Significant microwave absorption peaks and hysteresis effects were observed in low magnetic fields (30-500 Gauss), indicating different phases: Meissner phase, vortex glass phase, and normal state. The rapid disappearance of the Low-Field Microwave Absorption (LFMA) peaks with sample rotation, followed by a prolonged “memory” effect, was noted, suggesting the presence of superconducting vortices. A critical transition temperature of around 250K was identified.
To theoretically simulate these magnetic field-regulated transitions, a quasi-one-dimensional ladder model was used, representing randomly oriented one-dimensional superconducting channels in CSLA. The system transitions from a Meissner insulator state to a vortex glass state with increasing magnetic flux, showing different circulating current patterns. The memory effect observed experimentally was successfully replicated by stimulating a ring current in the middle of the ladder, modeling the slow “creeping” diffusion process of vortices.
This study provides strong evidence for the superconducting mechanism of CSLA at room temperature and demonstrates the complex phase transition behavior in quasi-one-dimensional strongly correlated systems under microwave excitation.
Collected Questions and Answers on Copper Substituted Lead Phosphate’s Research
Q1: How is the positive peak of low-field microwave absorption, different from the negative peaks in magnetic materials, determined?
A1: The determination is based on the reference signal from copper oxide-based free radicals measured simultaneously. The EPR signal of these radicals is strong, showing a clear negative peak at about 3350 Gauss. This reference peak helps determine the positive or negative nature of the low-field absorption peaks, indicating whether the absorption strength increases or decreases with the magnetic field.
Q2: Why choose X-band microwaves for detection? Does frequency impact the results?
A2: X-band microwaves (9.6GHz) are chosen for their high sensitivity in detecting superconducting states and their resonance potential with the superconducting gap. Higher frequencies could also detect superconductivity but require different equipment, making the experiments more complex.
Q3: How was the lattice gauge theory model established, and how well does it match experimental results?
A3: The model, a quasi-one-dimensional dual-chain ladder network, reflects the sample’s conductive channels. It models the transition from a Meissner insulator state to a vortex glass state with increasing magnetic flux, aligning well with the experimental findings.
Q4: What are the differences between the Meissner phase and the vortex glass phase, and how do they correspond to experimental observations?
A4: The Meissner phase is insulating with a chiral current but no local vortex current, while the vortex glass phase has local vortex currents. These phases explain the low-field saturated absorption and hysteresis effects observed in experiments.
Q5: How was the critical magnetic field and the coherence length of the sample determined?
A5: The critical field was estimated by aligning theoretical calculations with the experimental critical field of about 500 Gauss, reflecting the coherence length of the superconducting electrons.
Q6: What evidence supports the hypothesis that the sample is a 1D superconductor?
A6: Evidence includes the synthesis method, XRD characterization, anisotropic responses in magnetic levitation videos, and microwave absorption results.
Q7: Why does the EPR signal disappear when the magnetic field is rotated in the vortex glass phase?
A7: In the vortex glass phase, the randomly distributed vortices create a weak overall magnetization. Rotating the magnetic field direction disrupts this, leading to the disappearance of the EPR signal until the magnetic interactions among vortices readjust over time.